What is a black hole?
Black holes are certainly one of the most bizarre objects that can be found in our universe. Despite of the fact that the term black hole is probably as common as for example a planet, it is not exactly easy to tell what a black hole really is in a short, understandable and accurate way. A very often used definition draws a black hole as a volume of space where gravity is so strong that nothing, not even light, can escape from it. That certainly is true, but there is more to that and in what follows we try to point out some interesting features that are not explained so often.
The very first idea of an object that would not let light go first came from John Michell, an English country parson, in 1783. If we have a gravitating cosmic body, say the Earth, we can ask how fast do we have to throw a small object in the upward direction in order for the object to escape to infinity (or at least very far away) and not return. This is a classical high-school exercise of finding the escape velocity from an object of mass M and radius R that is v=√(2GM/R). In the case of the Earth we recover a well known value of 11.2 km/s. Now, from the simple formula for the escape velocity we see, that for an object that is either sufficiently heavy or has sufficiently small radius the resulting velocity can be made larger than the speed of light. Nature tells us that nothing can travel faster than light, so not only that such an object could not be seen, but there is no way anything else can escape from it because its strong gravitational force. The question remained whether objects like that really existed. Taking our Sun as an example, it would have to be more than 500 times in size, assuming the same average density, in order for the escape velocity from its surface to exceed the speed of light. This concept made only a little impression at Michell's time, but came back in 1916 when Albert Einstein formulated principles of general relativity and Karl Schwarzschild found the first solution to Einstein's equations.
Einstein showed us how to think about space and time. We usually do it separately: for us space is one thing and time is something else which exists independently on space and on what is happening in it. Such notion perfectly corresponds to our everyday experience. But according to Einstein's relativity, we can only think like that in the low speed limit. Once things started to be more extreme, we would notice that our normal laws of physics would not hold and in order to keep the laws the same form, we are forced to link space and time into one and single concept that is since than called spacetime. In addition, this spacetime is affected by presence of matter and the action is mutual. Matter deforms spacetime and the deformed spacetime tells the matter how to move in it. Newton's concept of gravitational force becomes unnecessary.
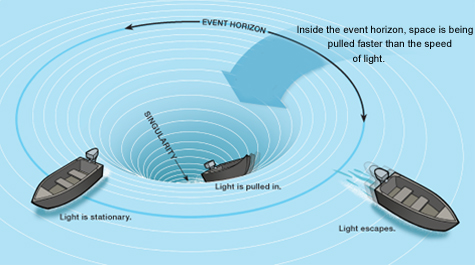
Let's focus on black holes now. When astronomers speak about them, they often make an unintentional impression that they are some kind solid objects. They are not. A black hole is a spacetime singularity that is enclosed by an event horizon. Both things are quite weird, but none of them is anything solid. The event horizon is what we usually thing is the black hole surface. In fact it is just a special place in a completely empty space, where spacetime is so extremely deformed by accumulated mass that it only allows one direction of motion – down into the singularity. Everything that reaches this point is forced to continue further down closer to the singularity which is hidden at the center of the event horizon sphere. The singularity is probably also nothing solid. We say probably because nobody knows. We do not have a good theory to describe it, we cannot probe it and event if we managed to send a robot to a black hole, there would be no way for its signals to penetrate the event horizon from the inside and reach us. Therefore, astronomers do not care about the singularity or about what is inside the event horizon sphere. We let that worries to string theorists to dream about. Astronomers only care about what is outside of the event horizon, what they can observe.
To stress the point once again, black holes are not like classical objects. There is no surface anywhere. It is just the quality of space and time, which both behave in a peculiar way close to a strong concentration of energy in the singularity, that we call a black hole.
Black holes are also one of the most simple objects in the universe we know. Imagine the Sun. It is merely impossible to describe it accurately. It contains about 1057 particles. For each of them we would need to record its position, velocity, quantum state, and so on. Then there are about as many photons with different energies and momenta. It all moves, interacts an evolves. No computer exists that would be capable of holding such an amount of information. A black hole, on the other hand, is like a single elementary particle. All the complexity of its parent star has been erased during the process of gravitational collapse and what has been left is an object that can be fully and completely characterized by three numbers only. They are the mass, rotation and the electric charge. In astronomy, it is common to forget about electric charge because we see that on average everything is electrically neutral in the universe and we believe that also black holes acquire as much positive charges as negative ones and they net electric charge is very very small if any. Hence we are left with mass and rotation only.
Mass is the less interesting quantity of the two. Mass is simply a scaling factor and it tells you whether the black hole is bigger or smaller, but without anything else around you would not be able to recognize any difference in spacetime distortion being two times further away from a twice as heavy black hole. It plays a role, however, if there is mass around the black hole.
Rotation is more special. First of all it may seem strange to talk about rotation in case of something that is only some distortion of spacetime rather than a real object. But the black hole was created from something. If that was a star, for sure it was rotating before it turned into the black hole. But from school we remember that there are certain universal conservation laws in the nature. The most basic ones that have to do with fundamental symmetries of space and time are conservation of energy and conservation of angular momentum. If the original star rotated, its angular momentum could not just disappear, it had to conserve. Indeed, it is in the singularity, we cannot reach it, but we know about it because it affects the surrounding spacetime structure. Astronomers often make a shortcut and say a black hole rotates rapidly. By that statement they mean that there is a high amount of angular momentum in the singularity hidden under the event horizon that strongly affects the spacetime outside. Surprisingly, there is a maximum limit on the amount of angular momentum the black hole can have. The more angular momentum, the smaller is the event horizon sphere. If the angular momentum stored inside the black hole reached certain value, the event horizon would disappear uncovering the singularity that would become exposed to the word. Such a situation is called a naked singularity and because it would cause a lot of trouble we think nature prevents it to happen. We call this principle cosmic censorship. In fact, the argument is more involved than simply postulate that it cannot happen because it is not convenient, for instance it would take an infinite amount of time to spin up a black hole and reach the maximal value. For us the important thing is the existence of maximal value of the contained angular momentum. Astronomers usually take that value as a scaling factor and measure the angular momentum of black holes in the units of their respective maximal value (it depends on mass). Such measure is then called spin and it can have value from zero (for a non-rotating black hole) to one (spin greater than one belongs to naked singularities).
A number of interesting things happen close to the horizon. One thing you would notice is that Euclidean geometry does not apply. If you measured circumference of two nearby circular orbits and divided both measurements by 2π to recover their respective radii, you would find out that the difference in the two radii measured that way is less than the difference in radial distances that is measured directly e.g. using light signal sent between the two orbits. A similar feature applies to time interval measurements. Time intervals that are measured at smaller radii (orbits closer to the black hole) appear to last longer than intervals measured by the same technique further away. Both effects are a consequences of distortion of both space and time due to strong gravity.